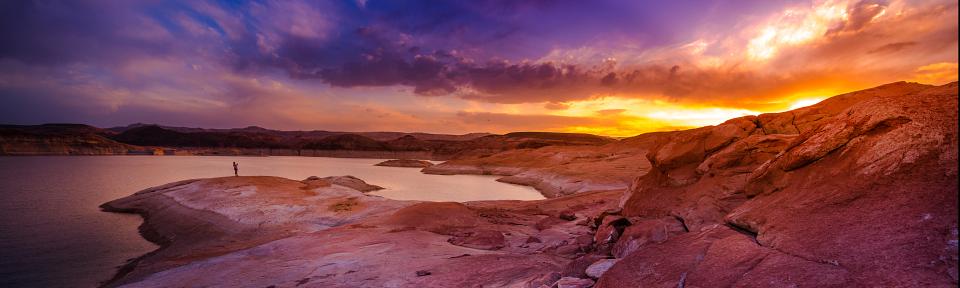
Shiu Lab
Shiu Research Lab
The Vascular Access & Health Laboratory at University of Utah School of Medicine and Salt Lake City VA Medical Center integrates medicine, bioscience and engineering to enhance dialysis access and reduce the burdens of chronical kidney disease (CKD), cardiovascular disease (CVD), and peripheral vascular disease (PVD). Our group uses a combination of experimental and computational techniques to conduct both clinical and translation research related to (i) hemodialysis vascular access, (ii) vascular dysfunctions and pathomechanics in patients with CKD, CVD and PVD, and (iii) mechanobiology.
We have received research funding from the National Institutes of Health (R01), Department of Veterans Affairs (Merit Review), Western Institute for Biomedical (Veterans) Research, American Heart Association, National Kidney Foundation of Utah & Idaho, other funding agencies, and the industry.